A Nose for Discovery
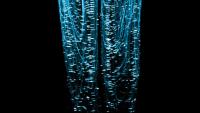
By Christina Hernandez Sherwood and Susan Conova, Photographs by Jörg Meyer
Just over 20 years ago, Richard Axel, MD, and his former postdoc Linda Buck, PhD, were awarded a Nobel Prize for discovering the human genes dedicated to producing all the odor receptors in our nose, as well as the organization of the olfactory system.
In his Nobel lecture, Dr. Axel explained why the olfactory system was such a ripe area of study.
“All organisms have evolved a mechanism to recognize sensory information in the environment and transmit this information to the brain where it then must be processed to create an internal representation of the external world. ... Biological reality, I argue, therefore reflects the particular representation of the external world that a brain is able to build, and a brain builds with genes.
If our genes are indeed the arbiters of what we perceive from the outside world, then it follows that an understanding of the function of these genes could provide insight into how the external world is represented in our brain.”
Drs. Axel’s and Buck’s discovery of 1,200 mouse genes dedicated to the detection of odor molecules— and 400 human genes, about 3% of our genome—opened the door to understanding how the external world of odors is constructed in our brain. And how that representation affects our actions and behaviors.
It also influenced investigations in even more diverse areas of biology. As a primal sense that alerts mammals to dangerous predators, olfaction offers a powerful model for investigating the curious transmission of trauma from one generation to the next. And as home to the largest gene family in the human genome, the nose furnishes a rich research landscape for understanding gene regulation throughout the brain.
Some 80 scientists, including two Nobel winners, count themselves alumni of Dr. Axel’s lab.
Neuroscientist Bianca Jones Marlin, PhD, Herbert and Florence Irving Assistant Professor of Cell Research in the departments of Psychology and Neuroscience and the Zuckerman Institute, is among them.
Dr. Marlin had been influenced by her early experiences as a teacher and was driven by curiosity about parental behavior and environmental factors that shape learning. She brought these interests to Dr. Axel’s lab … then took them in an entirely new direction.
Tracking Trauma
Dr. Marlin has long been asking questions about why people behave the way they do. During graduate school at New York University, that focus led her to look at how a mother’s brain adapts to care for her newborn. “When a mouse mom hears the sound of a mouse pup crying, she’ll turn toward the sound and pick up the pup,” Dr. Marlin says. “When a virgin mouse, who has never given birth, hears a pup crying, she usually ignores it.” Or worse, eats it.
Dr. Marlin found that oxytocin, which surges in the mom after birth, changes the way neurons in the auditory cortex of her brain respond to a pup’s sounds. And that changes her behavior. “We can even give oxytocin to a virgin mouse, and the mouse will act more maternally toward the pups, and her brain will show a mother’s signature of neural responses,” Dr. Marlin says. The research was published in Nature, and for her dissertation work, Dr. Marlin received the Donald B. Lindsley Prize in Behavioral Neuroscience from the Society for Neuroscience, which cited the potential of the research to increase our understanding of how vital bonds between parent and offspring form and sometimes fail.
For her postdoc, Dr. Marlin wanted to switch gears. Other researchers were finding in mice that offspring could inherit traumas experienced by their parents, not through genetic mutations or changes in parenting style, but potentially through mysterious epigenetic changes.
Epigenetics refers to biochemical markers, acquired from experiences or environmental conditions, that become attached to genes and can change the way those genes are expressed. Identical twins, for example, have the same genes, but their different experiences may change their gene expression.
Such marks are supposedly wiped clean when sperm meets egg. “So how can epigenetic changes be passed to offspring?” Dr. Marlin asks. “We wanted to find the mechanism.”
A Model System
This time, instead of looking at moms, Dr. Marlin’s research focuses on fathers. The students and post-docs in her lab working on this project affectionately call themselves “Team Dad.”
The “dad” starts out as a young male mouse in the lab. He sniffs a strong almond scent wafting through the air, then startles as a mild electric current in the floor of his cage reaches his feet. Over the course of just a few days, he learns that the almond smell will come with a mild shock, and whenever he senses the odor, he freezes.
The experiences permanently change the mouse’s sense of smell. Inside his nose, new epigenetic marks change the destiny of many odor-detecting cells. Stem cells originally programmed to produce neurons that detect other odors, such as mint or strawberry, are reprogrammed to produce more almond-sensing cells.
Here’s where things get weird. Ten days after the shock and odor, the male mouse mates with a “naive” female who has not experienced the same stressor. When the pups become young adults, their noses have the same heightened sensitivity to almond as their father—despite never experiencing the stressor or even meeting their father.
“A morphological change in the nose, that’s a big deal,” says Dr. Marlin. “Biology doesn’t waste energy on changing things unless they’re important.”
This inheritance could further our ability to understand which changes in the parental brain are learned, and which are biologically activated—a question of personal interest to Dr. Marlin, whose biological parents were also foster parents and who is now both a biological and foster mother herself. “It’s really a study between nature and nurture, the innate and the learned, the environment and the individual,” she says.
But how does the inheritance work? In “Team Dad’s” experiments, the male’s offspring are raised solely by the mothers to rule out any social interactions that may create learned changes in the offspring.
One recent finding, published as a reviewed preprint in eLife by Dr. Marlin’s lab, suggests a stable molecular signal causes the epigenetic changes in the father’s nose and encodes those physical changes in his sperm, ultimately affecting his offspring’s olfactory sensitivity.
It’s all part of answering the bigger question that her lab investigates: How do parents biologically pass down what they’ve learned and experienced to promote survival in the next generation?
Molecular Musings
Soon after Drs. Axel and Buck received their Nobel Prize in Sweden, Columbia professor of biological sciences Stuart Firestein, PhD, reflected on the impact of the groundbreaking work: “All at once, immense new possibilities opened up in the field of olfaction,” he wrote in an essay for the journal Neuron.
Such possibilities were enticing to rising investigators like Stavros Lomvardas, PhD’02, then a graduate student interested in gene regulation. Dr. Lomvardas found his scientific focus when a member of his thesis committee spoke up with “brilliant” comments and criticism. It was Dr. Axel.
“I started reading about his research, and I realized that he was working on a fascinating problem that could be something I could use my expertise on—a problem worth dedicating your life to,” says Dr. Lomvardas, now the Roy and Diana Vagelos Chair of Biochemistry and Molecular Biophysics and Herbert and Florence Irving Professor at the Zuckerman Institute. The aspiring scientist learned there was just one criterion for joining Dr. Axel’s lab, and it wasn’t publications or acclaim. “The only thing he cares about is whether the question you’re trying to answer is intellectually interesting. Of course, for something to reach a threshold of being interesting to Richard, it’s almost an impossible question.”
Though Dr. Axel’s research at that point had shown that each olfactory neuron expresses only one of the 2,400 odor receptor genes in the nose (the 1,200 genes inherited from mom and the 1,200 from dad), how each neuron made the selection was unknown.
An animal’s sense of smell relies on the one-neuron, one-receptor rule, which ensures that the response to an individual odor is the same each time the odor is encountered. Without that restriction, the brain simply could not make sense of the information it receives from the nose. Dr. Lomvardas wanted to elucidate the process, and he joined the lab as a postdoctoral trainee in 2002.
It took nearly two decades, but what Dr. Lomvardas found has far-reaching implications beyond solving a sticky problem in olfaction.
His studies reveal that several dozen regions scattered across the genome bend the chromosomes to bring multiple odor receptor genes together into interchromosomal clusters. This unusual interchromosomal clustering silences most of the olfactory receptor genes tangled in these genomic interactions, but also enables the assembly to activate nuclear hubs that facilitate the transcription of olfactory receptor genes that escaped silencing. Competition between the handful of activating hubs in each nucleus, mediated by the olfactory receptor RNA, results in eventual incapacitation of all but one of these hubs and transcriptional singularity. After this “winner takes all” process is complete, a feedback signal generated by the olfactory receptor protein stabilizes the singular olfactory receptor expression for the life of the neuron.
It’s a process that, biochemically and thermodynamically, seems impossible, Dr. Lomvardas explains. Somehow these bits of DNA on different chromosomes find each other in the vast, three-dimensional nucleus of the olfactory neuron. “It’s the equivalent of sitting at opposite ends of Times Square and throwing two quarters in the air,” Dr. Lomvardas says, “and the two quarters somehow meet each other.” His lab continues to work out more of the details of how the bits of DNA move toward each other and remain attached once they’ve reached a cluster.
Before this discovery, researchers thought that genes were largely regulated by other parts of the same chromosome. Dr. Lomvardas’ research revealed that gene regulation could be more complicated.
The olfactory system is the ideal backdrop for studying these questions because of the large number of genes there, Dr. Lomvardas says. But there are similar connections among chromosomes throughout the mammalian nervous system.
“All over the nervous system, both in mice and in humans, there is tremendous interconnectivity between chromosomes that follow similar principles,” Dr. Lomvardas says. “Even if olfactory neurons are an extreme example due to the number of genes involved, they are an extreme example of something that’s happening throughout the nervous system and believed to have regulatory roles in generating the diversity of the nervous system.” To achieve stable and specific connectivity between chromosomes, neurons have had to evolve novel biochemical principles, as described in a publication from the Lomvardas lab recently accepted to the journal Nature.
The work also has important implications for human health, including COVID-19 and some neurodegenerative disorders. Consider the case of anosmia, loss of the sense of smell. In 2022, Dr. Lomvardas and co-authors described in the journal Cell how the cascade of immune response in COVID-19 disrupts chromosomal interactions in olfactory neurons— even though the neurons aren’t themselves infected by SARS-CoV-2. “We’re following up on that to determine the trigger for this massive change in nuclear organization,” he says. “We think long COVID and brain fog and changes in the nervous system might have to do with this effect of the infection on the organization of the nucleus.”
The early onset of Alzheimer’s disease is also sometimes accompanied by loss of the sense of smell. Dr. Lomvardas’ lab has found that, in some mouse models, chromosomal interactions become disordered early in Alzheimer’s disease, resulting in loss of olfactory receptor expression. “We think there are some commonalities between the immune responses in COVID-19 and the inflammatory responses reported in the progression of Alzheimer’s disease,” he says. “The olfactory system is the perfect place to look for these changes.”
The Power of Mentorship
As part of the next generation, Dr. Marlin’s own students are now taking the research into even more directions.
For instance, after discovering that a combination of shock and odor changes the fate of cells not only in a mouse father’s nose, but also in his offspring, Dr. Marlin’s “Team Dad” is probing whether offspring are born with their father’s stress response or just primed to learn to respond to the potential stressor more quickly.
“Team Mom,” on the other hand, is exploring how the maternal brain—specifically the hearing and smell centers—changes over time, and how it differs from the virgin brain. Using a technique called iDISCO, researchers can easily image a mouse’s entire brain, while isolating cells that fire at particular moments. “We really are pioneering in this space where we can image the whole brain to see what it looks like when a mother hears her offspring cry,” says Dr. Marlin.
Dr. Marlin knows her work has broad implications for humans: for foster parents like herself and her parents, and also for survivors of trauma. Dr. Marlin cites the example of children of survivors of the Dutch Hunger Winter famine of 1944-45, who experienced higher rates of death and illnesses such as diabetes, cardiovascular disease, anxiety, depression, and schizophrenia. She sees her role as building a robust foundation of basic science to inform future clinical applications. “I have packaged it beautifully so the next person can take that step,” Dr. Marlin says. “We always work in community. It’s wrong for me to think I could do it all.”
Members of the Lomvardas lab continue to explore the mechanisms of interchromosomal interactions.
“While we have identified some of the players involved in creating stable connections between DNA segments from different chromosomes, we have no idea how these connections are formed,” Dr. Lomvardas says. There is no precedent, he adds, for the assembly of such widespread, stable, and specific interchromosomal interactions in any form of life, so uncovering the genomic and molecular choreography behind them is a major research goal. “As biomedical scientists, we are equally intrigued by the emerging realization that such interchromosomal contacts are not a peculiarity of the olfactory system, but a general mechanism deployed by neuronal subtypes across the human brain. That immediately raises the possibility that they regulate nuclear processes intimately connected to neurodevelopmental, cognitive, and neurodegenerative disorders.”
It’s this strong community of researchers that keeps Dr. Marlin at Columbia, and that drew Dr. Lomvardas back in 2014 after an eight-year stint at the University of California, San Francisco.
“Having Richard here, talking to him about what’s going on in the lab and having his insight, is priceless,” Dr. Lomvardas says. Twenty years ago, Dr. Axel regularly walked among the benches of his trainees, asking questions and sparking impromptu conversations as the junior scientists toiled at their projects.
“There is something special about just talking about science,” Dr. Lomvardas adds, “versus setting up an appointment where somebody comes prepared to present their data and has already formed a model in their head.”
Along with olfaction-related projects, Dr. Axel, who is also an investigator at the Howard Hughes Medical Institute, is now at work on an altogether different line of research. Along with, and inspired by, postdoctoral fellow Tessa Montague, PhD, he is investigating the ability of cuttlefish to change the color and texture of their skin to approximate their surroundings. This latest endeavor, Dr. Axel says, illustrates the interactive scientific environment at VP&S, especially the flow of ideas among senior researchers and junior colleagues. “We talk a great deal,” he says. “We consider each other’s thoughts, often in a deep and critical way, and feel free to express excitement or concern.”
Mentorship, Dr. Marlin says, is forever. “I can rely on Richard to go back and forth on our scientific ideas, as well as the other amazing faculty here,” she says. “It’s a dream I have for my students: that at some point we will be sitting as colleagues, and what they’ve learned from me, their peers, their mentees, their new environments, will all come together to make their science something that changes the world.”